Electrons in cuprates follow the old law
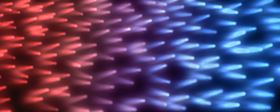
Long before researchers discovered the electron and its role in generating electrical current, they knew about electricity and were exploring its potential. One thing they learned early on was that metals were great conductors of both electricity and heat.
And in 1853, two scientists showed that those two admirable properties of metals were somehow related. At any given temperature, the ratio of electronic conductivity to thermal conductivity was roughly the same in any metal they tested.
This so-called Wiedemann-Franz law has held ever since – except in quantum materials, where electrons stop behaving as individual particles and glom together into a sort of electron soup. Experimental measurements have indicated that the 170-year-old law breaks down in these quantum materials, and by quite a bit.
Now, a theoretical argument put forth by physicists at the US Department of Energy’s SLAC National Accelerator Laboratory, Stanford University and the University of Illinois suggests that the law should, in fact, approximately hold for one type of quantum material – the copper oxide superconductors, or cuprates, that conduct electricity with no loss at relatively high temperatures.
In a paper in Science, the physicists propose that the Wiedemann-Franz law should still roughly hold if one considers only the electrons in cuprates. They suggest that other factors, such as vibrations in the material’s atomic latticework, must account for experimental results that make it look like the law does not apply.
This surprising result is important for understanding unconventional superconductors and other quantum materials, said Wen Wang, lead author of the paper and a PhD student with the Stanford Institute for Materials and Energy Sciences (SIMES) at SLAC.
“The original law was developed for materials where electrons interact with each other weakly and behave like little balls that bounce off defects in the material’s lattice,” Wang said. “We wanted to test the law theoretically in systems where neither of these things was true.”
Superconducting materials, which carry electric current without resistance, were discovered in 1911. But they operated at such extremely low temperatures that their usefulness was quite limited.
That changed in 1986, when the first family of so-called high-temperature or unconventional superconductors – the cuprates – was discovered. Although cuprates still require extremely cold conditions to work their magic, their discovery raised hopes that superconductors could someday work at much closer to room temperature – making revolutionary technologies like no-loss power lines possible.
After nearly four decades of research, that goal is still elusive, although a lot of progress has been made in understanding the conditions in which superconducting states flip in and out of existence.
Theoretical studies, performed with the help of powerful supercomputers, have been essential for interpreting the results of experiments on these materials and for understanding and predicting phenomena that are out of experimental reach.
For this study, the SIMES team ran simulations based on what’s known as the Hubbard model, which has become an essential tool for simulating and describing systems where electrons stop acting independently and join forces to produce unexpected phenomena.
Their results show that when you only take electron transport into account, the ratio of electronic conductivity to thermal conductivity approaches what the Wiedemann-Franz law predicts. “So, the discrepancies that have been seen in experiments should be coming from other things like phonons, or lattice vibrations, that are not in the Hubbard model,” Wang said.
SIMES staff scientist and paper co-author Brian Moritz said that although the study did not investigate how vibrations cause the discrepancies, “somehow the system still knows that there is this correspondence between charge and heat transport amongst the electrons. That was the most surprising result”.
From here, he added, “maybe we can peel the onion to understand a little bit more”.
This story is adapted from material from the SLAC National Accelerator Laboratory, with editorial changes made by Materials Today. The views expressed in this article do not necessarily represent those of Elsevier. Link to original source.
Source link